Atomic Spectroscopy:
Atomic spectroscopy is the technique for determining the presence and concentration of an elemental analyte by its electromagnetic or mass spectrum. It is increasingly applied as part of impurity analysis of products and package systems as referenced in USP chapters and their EP counterparts such as <232 / 233>, <661.1 / 661.2>, <381>, and much more. Several analytical techniques, or methodologies, exist, and an understanding of their relative performance and limitations is critical in achieving accurate and reliable real-world results.
There are three widely accepted analytical technologies for atomic spectroscopy – atomic absorption, atomic emission, and mass spectrometry. The most common types of instruments incorporating these technologies include:
- Flame Atomic Absorption Spectroscopy (Flame AA)
- Graphite Furnace Atomic Absorption Spectroscopy (Graphite AA)
- Inductively Coupled Plasma Optical Emission Spectroscopy. (ICP-OES)
- Inductively Coupled Plasma Mass Spectrometry (ICP-MS)
The operating principle, potential benefits, and disadvantages of each major technology will be outlined below.
An Overview of Atomic Absorption (AA) Spectroscopy:
Atomic Absorption (AA) refers to the phenomenon in which electrons in a ground state atom absorb light energy and reach an excited state, moving to a higher energy level. Generally, different atoms (elements) are excited by different wavelengths of light. By passing light at the appropriate wavelength through an atomized sample, the amount of energy absorbed at the wavelength corresponding to the element of interest (analyte) can be measured. As concentration of the analyte increases, the amount of light energy absorbed at this wavelength will increase. This relationship between energy absorption and the concentration of analyte can be used to determine unknown sample concentrations by interpolation based on measurements of known standards.
Performing atomic absorption spectroscopy requires the following:
- A primary light source
- An atomizer / atom source, which breaks the sample down into its individual elements
- A monochromator to isolate the specific wavelength of light to be measured
- A detector to measure the light accurately
- Electronics to process the data signal
- A display or software to interpret and report the results
The general sequence of steps for Atomic Absorption are as follows, with additional considerations depending on the type of AA technology used.
A lamp is installed in the AA unit. Generally, the light source is a hollow cathode lamp (HCL) or an electrodeless discharge lamp (EDL), with different lamps being used for different analytes (elements of interest). Since different elements are excited by different wavelengths of light, lamps able to emit light at the desired wavelength must be used. In some cases, a few elements may be combined in a multi-element lamp.
The liquid sample is nebulized and sprayed into an atomizer, or atom source. In AA, the source of energy for free-atom production is heat, though as discussed below, different approaches to this are available. The heat source drys the sample and atomizes it, breaking it down into individual elements. The light at a certain wavelength is passed through the atomized sample, where it is absorbed by elements of interest, and that energy absorption is measured.
Flame Atomic Absorption Spectroscopy:
In flame AA, the atomizer, or atom source, is most commonly in the form of an air/acetylene or nitrous oxide/acetylene flame. The sample is introduced as an aerosol into the flame, which is aligned so that the light beam passes through the flame, where the light is absorbed, measured, and reported by the instrument. While flame AA is rather ubiquitous and inexpensive relative to other technologies, imprecise atomization, sample retention, and beam pathways yield less sensitivity than technologies described below.
Graphite Furnace Atomic Absorption Spectroscopy:
In comparison to flame AA, Graphite Furnace Atomic Absorption (GFAA), the sample is introduced directly into a graphite tube. The tube is then heated according to a programmed series of steps to remove liquid solvent and to atomize the remaining sample. Because the sample is introduced to the tube, and not a spray chamber as with flame AA, the entire sample is atomized, and the atoms are retained within the tube for an extended period. Light is passed through this tube and absorbance measured. As a result of this improved analyte control relative to flame AA, sensitivity and detection limits are significantly improved.
However, graphite furnace analysis times are longer than those for flame AA. In addition, fewer elements can be determined using GFAA based on atomization programs. However, the enhanced sensitivity of GFAA, and its ability to analyze very small samples, significantly expands the capabilities of atomic absorption as an analytical technique.
Inductively Coupled Plasma Optical Emission Spectroscopy (ICP-OES):
Plasma: a gaseous charged ions phase of matter containing
Generation of Plasma: RF is applied to a coil generating a magnetic field that causes collision with electrons and argon atoms that generate argon ions. Argon reaches a temp of 10,000 K.
Application of Plasma:
Energy Source
Break bonds of molecules to atomize sample
Introduce energy for electron promotion.
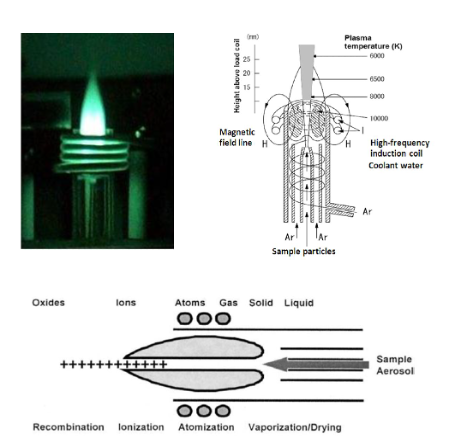
Whereas AA measures the amount of light of a certain wavelength absorbed as certain elemental atoms enter an excited state, inductively Coupled Plasma Optical Emission Spectroscopy (ICP-OES) is the measurement of the light emitted by the elements in a sample introduced into an ICP (Inductively Coupled Plasma) source.
The sample solution is introduced to the instrument by a pump, where it is nebulized. The resulting aerosol is injected into an argon-plasma with a temperature of 6000-8000 K. When a sample passes through the Inductively Coupled Plasma, it is atomized, ionized, and energized. The resulting elemental ions, being in an energized state, are inherently unstable. As the atom returns to its ground state, and electrons return to their stable energy level, the energy is released in the form of light. This light emission is passed through a spectrometer, where it is split by wavelength, before reaching a detector. As each element has a typical emission “fingerprint”, ICP-OES can identify and quantify all elements present in a matrix. Similar to the absorption concept in AA, the measured emission intensities from an ICP-OES analysis are then compared to the intensities of standards of known concentration to obtain the elemental concentrations in the unknown sample.
ICP-OES is substantially more complex than AA, leading to increased cost and analysis time, but represents a considerable improvement with respect to sensitivity. Additionally, because a light source at a specific wavelength is not required, all elements can be assessed concurrently, versus one at a time with traditional AA.
Induction Coupled Plasma Mass Spectrometry:
ICP-MS differs from ICP-OES with respect to the type of measurement and detection system used. As a sample is atomized and ionized by the plasma source, the charged ions are passed into a mass spectrometer, where ions are split according to their mass before reaching the detector, which in turn identifies and quantifies the elements present in a sample based on their mass. This approach to measurement yield a significant improvement in sensitivity even relative to ICP-OES
Detection Limits and Measurement Range:
The relative measurement range and detection limits of atomic spectroscopy technologies are summarized below.
Atomic Absorption
- Techniques have different ranges based on flame or graphite furnace
- Flame: few hundred ppb to a few hundred ppm
- Furnace: mid ppt range to few hundred ppb range
ICP-OES
- Two different observation modes: Axial and Radial, with about 20x difference between Axial and Radial
- High ppt to mid % (parts per hundred)
ICP-MS
- Most sensitive and widest range
- Range is a few ppq to a few hundred ppm
- Higher concentration samples can be diluted to reach operating range of the instrument
Analysis Speed & Limit:
The relative speed vs detection limit of atomic spectroscopy technologies is summarized below:
Atomic Absorption
- Liquid samples are desolvated, vaporized, and atomized by a flame or a graphite furnace.
- The gaseous atoms pass through a beam of light emitted from a radiation source such as a Hollow Cathode Lamp.
- The element absorbs an amount of light at a certain wavelength that is proportional to its concentration. The process is repeated for each element, and may require different light sources for detection.
ICP-OES
- Liquid samples are desolvated, vaporized, atomized and excited in an argon plasma.
- Excited atoms relax back to ground state and emit characteristic wavelengths of light, the intensity of which is proportional to its concentration
- A detector collects all the emitted wavelength of light in a single replicate.
ICP-MS
- Liquid samples are desolvated, vaporized, atomized and ionized in an argon plasma
- Elements have different mass once ionized, with each element forming its own characteristic mass spectrum.
- An ion counting detector counts the ions at each mass-to-charge ratio which is proportional to the concentration of each element.
Performance vs. Investment of each Technique:
The relative performance, from the standpoint of sensitivity, relative to cost of investment is summarized below.
Atomic Absorption Consumables
- Flame uses Lamps, Gases & O-rings
- Furnace in addition need Graphite tube and Caps & Holders
ICP-OES Consumables
- Argon gas
- Glassware: torches, spray chambers, nebulizers
ICP-MS Consumables
- Argon gas
- Glassware: torches, spray chambers, nebulizers
- Cones: Skimmer and Sampling
Considering detection limit, speed of analysis and overall instrument / technique performance, ICP-MS is most versatile atomic spectroscopy technique for determination of elemental impurities in drug product, components, and packaging components. Its ability to assess multiple elements concurrently, and to levels of sensitivity required for even the most stringent test limits, makes it especially useful as a tool for impurity analysis to fulfill regulatory requirements such as those established in USP <232 / 233>, <661.1 / 661.2>, <381>, and much more.
The CS Analytical Team are experts when it comes to your metals testing requirements especially as they relate to container and package system qualification requirements. We can help you define the most optimal approach and technique to ensure all regulatory requirements are met when it comes to metal content analysis.